In 2018 we are launching the Translational Institute of Medicine (TIME) at Queen’s, beginning in the Department of Medicine. Like all good ideas TIME was born from a conversation over coffee. Stephen Vanner and I were trying to figure out how to grow the research pie, train more researchers and ensure that all faculty had access to research resources, which sometimes are hidden in plain sight. This blog will be your introduction to TIME. Although we have been hard at work on building TIME for almost 2 years, formal approval for several key components is pending, so consider this a preview. TIME has 4 components:
- A new graduate educational program
- New state-of the art infrastructure
- A new on-line, virtual community tool (Uniweb®) to connect researchers with collaborators and to locate and access both new and existing infrastructure
- A Team of scientists and clinician-scientists (who are TIME’s engine).
Dr. Stephen Vanner will be the inaugural Director for TIME and Dr. Paula James will lead the Translational Medicine Graduate Program. TIME is ably supported at the administrative level by Ms. Whitney Montgomery. TIME will be a Faculty of Health Sciences-based institute (once approved by senate). Administratively, TIME is based in the Department of Medicine (DOM) and its funding currently derives from our physicians. The goal of creating TIME is to enhance research in the DOM (and beyond), and to train the next generation of researchers in this approach to discovery and the advancement of human health. TIME is also envisioned to enhance connections-amongst faculty, and from faculty to crucial research platforms and resources.
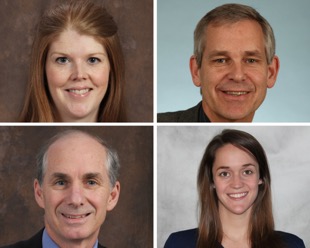
Clockwise from top left: Dr. Paula James, Dr. Stephen Archer, Ms. Whitney Montgomery
and Dr. Stephen Vanner
When we present the TIME concept people are generally excited. They sense that there is a certain practicality to approaching human disease from multiple levels, harnessing basic science, preclinical research, clinical trials, and population health to understand human disease and improve human health. However, many ask, what do you mean by “Translation”. It is appropriate to start with a definition of the word translation. To this end I consulted the Oxford English Dictionary (OED). While not the first English language dictionary, the OED was the first to use crowd-sourcing (the wisdom of the masses) to create and continuously update its definitions. The OED relies on how words are actually used in society to shape definitions.
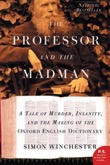
First published on February 1, 1884, the OED represented a radical departure from the prior methods of creating definitions. Before OED definitions relied on the opinion of a single learned source (person). In creating the OED James Murray put a call out worldwide for definitions of words, each to be accompanied by an illustration of their use in a sentence. These were them compiled, adjudicated and the rest is history! For more on this, I recommend Simon Winchester’s, The Professor and the Madman . In a nutshell, the OED defines by example. Let’s see what the OED says about translation, as it applies to Science and Medicine. Definition: Translation: The conversion of something from one form or medium into another. The OED offers several examples of the use of translation that are relevant to this blog, including:
- ‘The translation of research findings into clinical practice’
- ‘However, translation of genomic research discoveries to improved clinical outcomes can occur only with an informed professional workforce.’
- ‘The lack of capacity for research will stop the translation of discoveries in basic science into clinical practice.’
Canada’s major funding agency CIHR acknowledges 4 types of research or research themes (see Table below). Table 1: CIHR Research Themes:
These themes are valid, but the table falls short of acknowledging that research and discovery, clinical care and population health are not discrete pillars; rather they are dynamic nodes that are meant to interact one with another. Translational research moves between themes with the goal of better understanding and improving human health. In this regard CIHR research themes are somewhat like electrons. They exist in a cloud and one cannot precisely pinpoint whether a research discovery (and thus the researchers involved) is primarily grounded in one theme or several. This is a riff on the Heisenberg Uncertainty Principle. Heisenberg noted that in Quantum Physics the position and the velocity of any object cannot both be precisely measured at the same time. 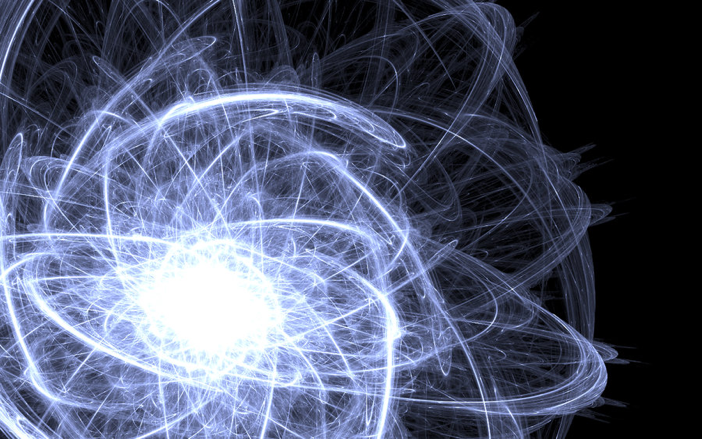
Representation of electron cloud
Likewise, in the translational continuum a discovery is always moving to and fro between bench, bedside and society. This leaves us with the uncomfortable truth that knowledge is always evolving. When we knock down silos and foster cross-themed research we can (sometimes) catalyze discovery. Translational researchers are the folks who buzz back and forth across the boundaries from Theme to Theme, they are akin to the bees that cross pollinate and make the honey! From time to time translational scientists, be they MD or PhD, basic or clinical, can move from theme to theme rapidly because they have the vision to see a path from a fundamental scientific observation to human health. They see how a bacterial product that breaks down thrombus might be a potential clot buster in patients with thrombotic heart attacks or strokes. Their vision leads to clinical trials, that assess safety and efficacy, and society reaps the benefit from drugs like tissue plasminogen activator (tPA) which are now used to treat strokes and heart attacks. Translational oriented research teams see the relationships between Genomics and disease. Goldstein and Brown recognized that patients dying of premature heart attacks have mutations in the low-density lipoprotein receptor (LDL-R) gene. This lead eventually to drugs, such as the statins, which lower LDL cholesterol and improve survival. 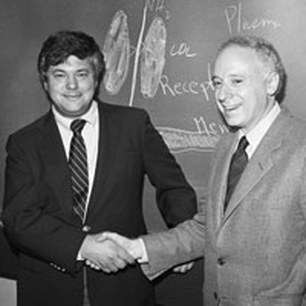
In most cases, the translator has the vision and a core area of expertise and enlists (or indirectly inspires) others (who have complementary expertise) to make the translational journey. Think of translational research as a relay race in which the baton is passed from scientists to physician and back. However, unlike a relay race, the runners many not always be part of one team, and the legs of the race are not equal in length, nor are they necessarily sequential. Perhaps translational research is more like an orchestra. The work of many, each with a unique role, translating the basics (notes on a page) into the applied, a beautiful symphony.
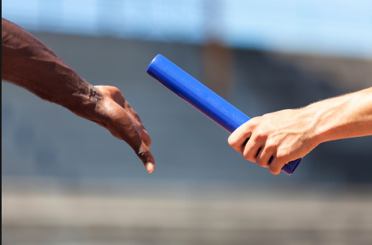
This is not to say that everyone should take a translational research approach. Not all research should focus on humans or human health (humans are but one of the earth's species and earth is but one planet in a vast universe). Not every question has a short or medium-term translational goal. Moreover, we need highly-focused basic scientists, who may have a laser-like focus on going deeply into an area of research (knowing a lot about a little). Those who ask and address curiosity-based questions not only increase knowledge but eventually their work may be understood to have relevance for human disease. Theether-a-go-go gene that makes fruit flies little legs shake when they are anesthetized encodes the same potassium channel (called HERG in humans) that makes humans experience sudden cardiac death through the Long QT-syndrome and a rhythm called torsades. Understanding this ion channel, identified in a fruit fly, allows us to test the many medicine we prescribe for patients drugs and ensure that they don’t interfere with HERG channel function and thereby screen out those drugs that are likely to cause long QT syndrome and put a person at risk of sudden death. Thank you fruit fly! 
A) HERG channel
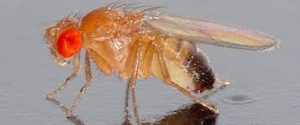
B) Drosophila (fruit fly)

C) Human long QT syndrome with Torsades de Pointes rhythm (sudden death)
Just as there is room in music for rap, classical and country genres, so too in science is there room for fundamental, as well as translational research. TIME, though proud of its mission, is not chauvinistic when considering other types of science. Now that we have a definition, let’s describe the pieces that comprise TIME. Led by Dr. Paula James, TIME’s Translational Medicine Graduate Program is anticipated to launch in Fall 2018, training Masters and Doctoral students. Students will be taught by basic scientists and physician scientists. A unique feature of this program is the exposure of students to patients, giving them greater insight into the phenotype of the diseases that they study, and enhancing the human impact of disease. The translational agenda will be advanced by the 30+ TIME instructors (Queen’s faculty who walk the translational walk in their research), and by TIME courses (which include patients and advance a Medical perspective that encourages a translational approach to research). In addition to the conventional research training (the interaction of the student and their principle investigator based in the lab), students will participate in a course called Profession of Medicine, led by Dr. Rachel Holden, engaging students in Medical Grand Rounds, bringing them closer to the “bedside”. Research Success Skills, led by Dr. Anne Ellis, will teach students the practical side of what they need to know to perform research, such as how to navigate the ethical and regulatory regulations that govern us and how to write a grant. They will learn about the molecular basis of diseases translational style, in a course called Translational Medicine, led by Drs. Mark Ormiston and Paula James. In this course, a lecture is followed by conversation with a person affected by the disease. Imagine the power of such a lecture. First, students hear about bleeding disorders and learn the molecular basis for bleeding disorders such as von Willebrand disease, hemophilia and more. Then they talk to a woman who has lived a life plagued by heavy menstrual periods because of undiagnosed von Willebrand disease. Her personal story of the disease burden and the relief that comes with receiving the correct diagnosis and a treatment (that is based on translational knowledge) is an unforgettable story, which has impact and motivates students and faculty alike. Lectures on the role of mutations in a gene called BMPR2, that promotes familial pulmonary arterial hypertension (PAH) or mitochondrial dynamics as a therapeutic target in PAH may seem a bit dry. Not so, when you then meet a patient with PAH who is on three classes of PAH-targeted drugs, costing more than $150,000/year in aggregate who still cannot walk without oxygen and faces a 5-year survival of only 50%. Suddenly, understanding how we might translate science to new therapies, whether by fixing the BMPR2 mutation or therapeutically targeting the enzymes that lead to disordered mitochondrial dynamics, becomes more relevant and compelling. In meeting the patient, our students will gain vision and perspective. In the parable of the blind man and the elephant, wise men grab isolated parts of the elephant and wrongly conclude the nature of the beast. 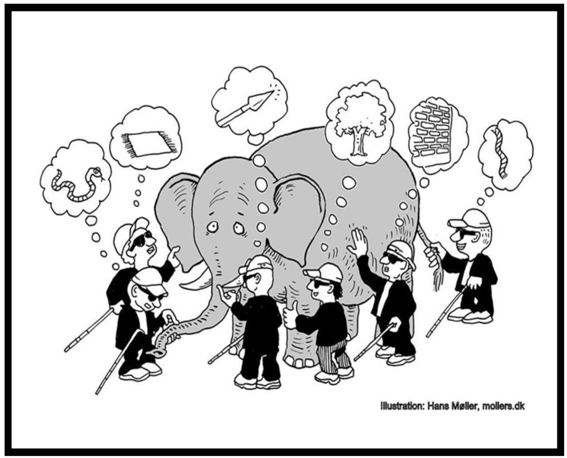
Parable of the blind man and the elephant: all were partially right and all partially wrong in their understanding of the beast they were dealing with.
By taking a translational approach, students gain vision and learn to see the totality of the problem, using a lens that focuses not only on patients but on molecules and populations. That doesn’t mean instant cure; but at least our graduate students will better understand the elephant in the room and better understand the patient phenotype. This better equips them to develop new diagnostic and therapeutic approaches. Translational Medicine graduate students will benefit from work in progress sessions, coming out of their labs and learning to appreciate and critique a range of scientific approaches that range from basic science to population health (the 4 CIHR themes). They will be exposed to faculty who have careers in diverse types of basic science and Medicine and thus be exposed to many role models for translational research, in addition to what they learn in their own laboratory. The close exposure to patients offers better access to cells, tissues and patient cohorts. It is a powerful motivator for success because it offers a constant reminder that the translational journey is not yet complete. Patients remind us that in 2018 we still need to better understand most diseases and that few diseases are cured (to the patients’ satisfaction). TIME also takes a new approach to infrastructure, recognizing that researchers need state of the art tools to be internationally competitive for scarce research funding monies (grants). Often at Queen’s University (and at all Universities I have visited) faculty may not know where existing research tools are located. In addition, truly revolutionary technologies are often too expensive and/or too complex to be run by individual investigators. TIME supports researchers and their students by electronically mapping “What’s Where” and by hiring expert scientists to operate these complex instruments and analyze the massive data sets they generate. TIME makes these resources available, on a cost recovery basis, and also trains students in these disciplines. TIME members (and others at Queen’s) will have access to several potent infrastructure resources including those in the recently opened Queen’s Cardiopulmonary Unit (QCPU) and Gastrointestinal Disease Research Unit (GIDRU).
Examples of infrastructure include, Next Gen sequencing (Illumina), A Cytof mass cytometer (Fluidigm), a micro PET-SPECT-CT scanner (MI Labs), a cell culture facility, and a 2-photon, super-resolution, intravital confocal microscope (Leica). QCPU is also unique in that it has spaces for trainees and a satellite clinical facility of the Kingston Health Sciences Centre.
The latter point means patients can be seen there, indeed a fully operational clinical echocardiography facility is housed within QCPU. Medicine and research are existing side by side, bringing patients to the researchers and vice versa. As unique as these expensive tools are, they would not be useful were it not for the scientists who run QCPU and operate these complex devices. Dr. Charlie Hindmarch directs the molecular and transcriptomics core whilst Dr. Patricia Lima operates the 2-photon confocal system. The TIME Uniweb site links 30 faculty who provide the Translational Medicine graduate program curriculum, the members of the DOM who fund TIME, as well as linking all these individuals to TIME infrastructure and potential collaborators (and their infrastructure) at Queen’s, regardless of home Department. Think of Uniweb (developed by an Ottawa based company) as an electronic Who’s Where; What’s Where app. Want to get flow cytometry done? Check on the TIME Uniweb site. Want to know who does transcriptomic research? Check the communities on the site, looking for people studying bleeding disorders or pulmonary hypertension at Queen’s.
Most importantly, TIME is a community of independent scientists, clinician-scientists, and clinicians from several Departments in the Faculty of Health Sciences. Each is focused on improving the understanding of human diseases and advancing diagnosis and therapy through research and discovery. Some are primarily physicians, such as Dr. Christine D’Arsigny, Director of the Pulmonary Hypertension Clinic at Kingston Health Sciences Centre (KHSC). They see the importance of TIME in creating new knowledge and of translating that knowledge as a means of improving care for their patients. Others are basic scientists, such as Dr. Mark Ormiston, PhD., recently recruited from Cambridge University. Mark is cross-appointed in Medicine, Surgery and DBMS. He is interested in the role of natural killer cells in the dysregulated angiogenesis that occurs in PAH. Finally, there are physician-scientists, such as Dr. Paula James, who see patients and run their own scientific research programs. By exposing students to all these translators, each participating in a unique stage in the translational journey from the Bench to Bedside, TIME hopes to better support existing translational scientists and physicians-scientists and to train a new type of scientist who will emerge from the program with a passion for improving human health through science.
I have asked a number of researchers in the DOM to provide a vignette of their translational research programs, in order to help illustrate what we mean by translation, OED style! These vignettes introduce some of our faculty, and foreshadow what our graduate students will be exposed to when they begin the Translational Medicine Graduate Program. There are 3 vignettes in this blog and more will appear in this series over the next few weeks.
Rachel Holden, Professor of Medicine (Nephrology)
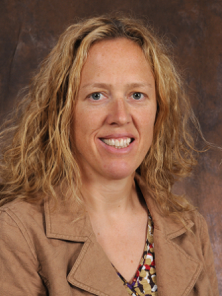
Big Picture Question: How can we prevent blood vessel calcification in patients with chronic kidney disease (CKD)? 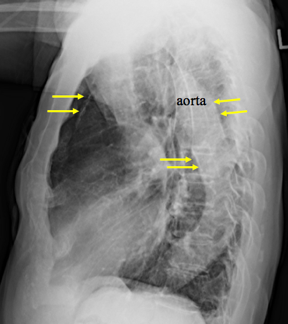
Patient with calcium in the wall of the aorta seen on Chest X-ray (so-called eggshell aorta)
The Goal: Vascular calcification is an active process in which vascular smooth muscle cells are transformed into osteoblast-like cells that express bone proteins that can cause mineralization of the vessel wall. Amazingly blood vessel cells are turning into bone creating cells! Vascular calcification often occurs in patients with poor kidney function (CKD) and is linked to abnormalities in calcium and phosphate metabolism. Paradoxically, vascular calcification is accompanied by alterations in bone turnover and increased fracture risk. We discovered that vitamin K is a key, modifiable risk factor for cardiovascular disease and bone fracture risk in patients with CKD. Vitamin K helps to prevent vascular calcification by interacting with matrix gla protein (MGP) and by enhancing bone health (e.g. through regulation of ). Our long-term goal is to develop one of the first treatments for vascular calcification. Defining the problem: At least 2 million Canadians have CKD, and over 20,000 Canadians require life-sustaining dialysis treatment for their disease. Their age-adjusted mortality rate is at least 10X higher than the general population. The severity of vascular calcification is one of the strongest predictors of mortality in this population. There are currently no available therapies to offer patients and indeed doctors are not advised by guidelines to go looking for this problem. The “Big Idea”: Vitamin K inhibits the progression of vascular calcification and prevents bone fractures in patients with chronic kidney disease based on its ability to increase the function of target proteins involved in blood vessel and bone health. Our idea is a novel strategy that seeks to address the mechanistic underpinnings of vascular calcification and bone fracture in patients with CKD. The Key Research Observation(s): MGP, a key inhibitor of vascular calcification, requires vitamin K to acquire calcium-binding activity. MGP becomes up-regulated adjacent to sites of calcification to limit the progression of calcification in a negative feedback manner. We have demonstrated marked up-regulation of MGP and down-regulation of critical vitamin K re-cycling enzymes, in the arteries of rats with CKD. This down-regulation of vitamin K metabolism may explain our previous observations that patients with low kidney function have very high levels of inactive vitamin K-dependent proteins in the circulation despite adequate Vitamin K dietary intake. Patients with CKD may therefore be at unique risk for biological consequences related to vitamin K deficiency. We were the first to demonstrate that a therapeutic dosage of warfarin, a vitamin K antagonist, globally depleted tissue vitamin K concentrations and markedly increased the susceptibility of vessels to vascular calcification in experimental chronic kidney disease. High dietary vitamin K on the other hand significantly increased tissue levels of vitamin K and reduced the incidence and severity of vascular calcification.
The availability of vitamin K to the vasculature may be critical to prevent vascular calcification in patients with CKD. Thus, we (a team of 2 scientists and key collaborators in the USA) have established the benefit of providing vitamin K to the vasculature in preclinical models. The Way Forward: Building upon this foundation of clinical and pre-clinical studies, we are actively conducting the iPACK-HD trial, a Phase II randomized, placebo-controlled clinical trial supported by CIHR, that is designed to determine whether vitamin K is superior to placebo in decreasing the progression of coronary artery calcification in patients with chronic kidney disease. In this multi-center trial we are also evaluating the impact of vitamin K treatment on bone fracture risk and body composition. Our goal is to develop a new strategy that will address the mechanistic underpinnings of vascular calcification and bone health in patients with CKD. The interested reader is referred to references at the end of this blog.
Charles Hindmarch PhD, Adjunct Professor Medicine (QCPU Scientist, Director Genomics, Transcriptomics and Molecular Medicine)
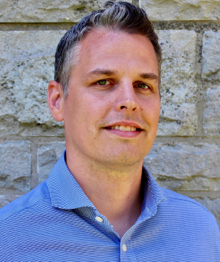
Big Picture Question: Can we use transcriptomics and genomics to advanced personalized medicine for pulmonary arterial hypertension (PAH) and explain why women are more affected by this disease? 1) The Goal: To use genomic and transcriptomic approaches to stratify patients with pulmonary arterial hypertension (PAH) with the aim of guiding better treatment strategies and the identification of novel therapeutic targets. 2) Defining the problem: Pulmonary arterial hypertension (PAH) is a disease that results in increased blood pressure in the arteries that supply blood to the lungs. PAH is a rare disease (<10,000 cases in Canada), but it contributes a significant burden to healthcare and is often fatal; PAH has a 50% mortality at 5-years in spite of increasing treatment costs. There are both idiopathic (meaning that the cause is unknown) and heritable (meaning there is a familial cause) forms of PAH. If you have a mutation in a gene called BMPR2, you are 10,000 times more likely to develop PAH than a member of the general population. We also know that the lung cells of people with PAH have dysfunctional mitochondria compared to healthy people; mitochondria are intracellular organelles that, in addition to generating energy, regulate cell proliferation and programmed cell death. As with all complex diseases, PAH is polygenic (regulated by many genes) and effects different people for different reasons and with differing severity. For example, only 1 in 10 PAH patients respond well to inexpensive vasodilatory drugs that relax blood vessel tone. These vasodilator responsive patients are mostly idiopathic patients and have a favorable outcome. In contrast, heritable PAH patients don’t respond well to these therapies. Several studies have identified that while PAH disproportionately affects women (4X more than men) paradoxically, women with PAH have better survival rates than do men. 3) The Big Idea: The aim of personalized medicine is to use some empirical measure in order to stratify patient groups appropriately in order to guide treatment or look for common molecular mechanisms that describe each subgroup of patients. Combining clinical and laboratory phenotyping with state-of-the-art genomic tools, will help address some of the challenges of treating PAH. 4) The Key Research Observation: We are now able to read the 3-billion or so nucleotides of the human genome of an individual and gain insight into not only their genome and the millions of variations that make them unique, but the ways in which this genome is expressed in different tissues and able to regulate itself through various epigenetic modifications. We have the power to perform these studies within various discrete tissues (for example blood, biopsy, sputum etc.) or even within single cells, or cell types. The time is right for us to design preclinical experiments that allows us effectively translate genomics into clinical practice. In PAH, a recent study by a multidisciplinary team of clinicians and basic researchers (including Dr. Archer, Queen’s University) demonstrated translational proof of concept. Microarrays (technologies that allow the simultaneous detection of tens of thousands of genes within a sample) were used to identify peripheral blood gene expression signatures in a small cohort of PAH patient and correlate these profiles to whether those patients were responsive to treatment with vasodilators, or not. Gene expression data from blood can therefore be used not only to stratify patient groups, but guide good clinical decisions, ultimately avoid unnecessary medication. This approach is not only consistent with the Choosing Wisely® campaign, which deals with reducing harm by rationally applying conventional therapeutics – but the ultimate refinement of it. While the ability to stratify patients is an excellent translation of pre-clinical studies in a bench-to-bedside manner, this type of data also opens us up the tantalizing prospect that we can take this data from bedside-back-to-bench; molecular signatures common to subsets of patients may shed new light on the pathogenesis and mechanism of PAH and may indicate novel therapeutics that can be tested. 5) The Way Forward: The Queen’s Cardio Pulmonary Unit has combined clinical and basic scientists within an environment rich with both technology and optimism; the TIME is right for us to bridge the interface between the laboratory and the clinic in PAH research. Patients participating in pre-clinical studies in QCPU will contribute to a rich biobank of tissues that will allow genomic and transcriptomic data to be generated. The Genomics, Transcriptomics and Molecular Medicine lab that I run, not only has the necessary ‘wet’ lab infrastructure required to perform these experiments, but through connection with the Centre for Advanced Computing, my lab has the computational power to analyze the data and place it into an appropriate biological context. I am particularly interested in studying the sex-paradox of PAH; why are women are more susceptible to PAH, but are also protected against the most lethal manifestations of the disease? I have proposed to build a PAH cohort in QCPU that matches pre-menopausal PAH patients with age-matched male PAH patients and healthy controls. I will initially use transcriptomics to ask specific questions about which genes are commonly or differently regulated as a function of biological sex within cells that mimic lung endothelia, and whether these genes correlate with clinical outcome. The end goal here is to dissect the molecular mechanisms that underlie the paradox so that we can better understand and treat PAH in both male and female patients.
Paula James MD, Professor of Medicine (Hematology)
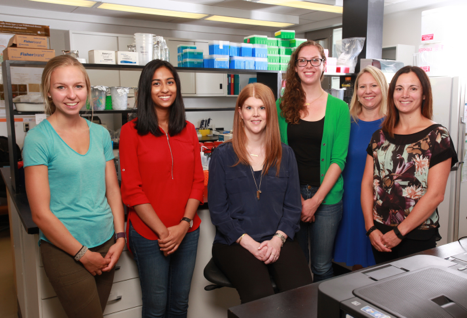
The James lab-Dr. James seated in centre
The Big Picture Question: What is the role of von Willebrand Factor (VWF) in angiodysplasia and abnormal bleeding in the bowels?
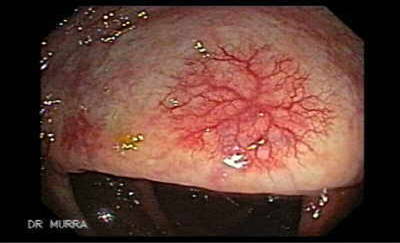
http://www.gastrointestinalatlas.com/english/colonic_angiodysplasia.html
The Goal: Our goal is to uncover the molecular mechanisms underlying the contribution of VWF to angiodysplasia so that we can optimize treatment for VWD patients who have gastrointestinal (GI) bleeding (blood loss from the gut due to formation of small leaky blood vessels like those in the picture above, taken from colonoscopy image). Defining the Problem: Von Willebrand disease (VWD) is the most common inherited bleeding disorder known in humans, occurring in 0.1% to 1% of the general population and affecting an estimated 35,000 Canadians. VWD is caused by quantitative or qualitative abnormalities of von Willebrand factor (VWF), a hemostatic multimeric glycoprotein that is synthesized and stored in endothelial cells and megakaryocytes. Beyond its established role in hemostasis and thrombosis (formation of blood clots), recent studies have identified a broader biologic activity for VWF, particularly endothelial VWF, in multiple processes including inflammation, cell proliferation and angiogenesis (formation of new blood vessels). A significant problem for ~ 20% of VWD patients is the presence of angiodysplasia in their intestines. Angiodysplasia arises from disordered angiogenesis and leads to friable blood vessels that can easily rupture resulting in hard to control bleeding, multiple hospital admissions, recurrent blood transfusions and exposure to unproven treatments of uncertain benefit. The Big Idea: Based on the recognition that VWD patients have increased rates of angiodysplasia compared to the general population, and the observation that patients with more severe forms of VWD are at particular risk, a few research groups started to focus on the idea that abnormal VWF could contribute to the development of angiodysplasia, and that interventions targeting VWF would normalize angiogenesis. Key Research Observation: In 2011, Dr. Anna Randi’s group at Imperial College London, published a seminal article that showed VWF to be a negative regulator of angiogenesis. In this paper, inhibition of VWF expression in endothelial cells by siRNA knock down caused increased in vitro angiogenesis and increased cell proliferation and migration1. In the James Lab, subsequent work by PhD candidate Soundarya Selvam (with TIME collaborators Dr. Stephen Archer, Dr. Mark Ormiston and Dr. Don Maurice), extended these observations and showed abnormal angiogenic behavior of BOECs (Blood Outgrowth Endothelial Cells) derived from VWD patients compared with controls2. These cells provide us with an easily accessible substitute for the cells lining the blood vessels (endothelial cells); which are hard to obtain from a patient! The Way Forward: This research has continued, using patient-derived BOECs to evaluate novel therapies for angiodysplasia (including atorvastatin, estrogen and VWF concentrates). The next experiments will involve using a mouse that is genetically engineered to lack VWF as a preclinical model to further understand the role of VWF in angiodysplasia to optimize treatment. The ultimate goal is to use this research to inform the design of a clinical trial in patients with VWD and angiodysplasia. Furthermore, outside the GI tract, vascular malformations also been described in the skin, nailbeds, uterus, prostate and nasal cavity of VWD patients and it is possible that this contributes to commonly seen bleeding such as epistaxis and heavy menstrual bleeding. Therefore, our research could be more broadly informative in terms of how to treat, or prevent, bleeding complications in VWD. For more about my lab visit here and for more about VWD visit here. The interested reader is referred to references at the end of this blog.
Of course, TIME does not exist in a vacuum. There are many established research centers and research Groups at Queen’s. TIME and these other groups/institutes are part of the research enterprise at Queen’s University within the Faculty of Heath Sciences. There is also the new Henderson Translational Research facility at KHSC. Like TIME, this new centre will make it easier for faculty and trainees to partner, to find infrastructure and to collaborate. 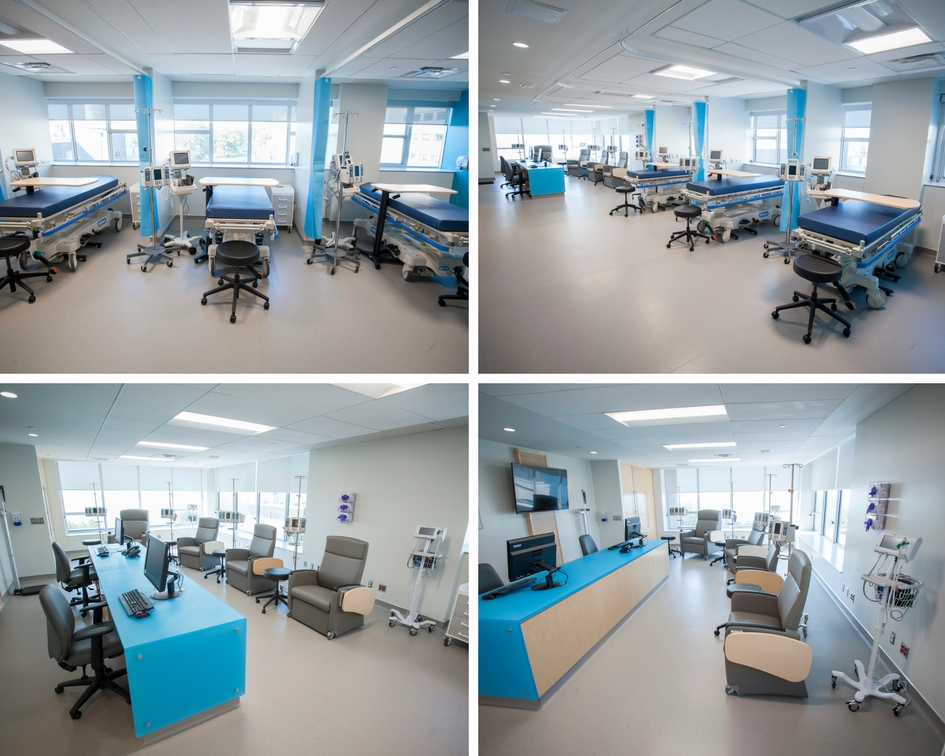
W.J. Henderson Centre for Patient Oriented Research - Clinical Investigation Unit
This is a first installment of translation research examples in the TIME domain. Stay tuned for upcoming blog posts on the work of Dr. Stephen Vanner (Gastroenterology), Dr. Yuka Asai (Dermatology), Dr. Mark Ormiston (Pulmonary Hypertension), Dr. Gord Boyd (Neurology) and me (the mighty mitochondria)!
Relevant references: Dr. Holden
- McCabe KM, Booth SL, Fu X, Shobeiri N, Pang JJ, Adams MA and Holden RM. Dietary vitamin K and therapeutic warfarin alter the susceptibility to vascular calcification in experimental chronic kidney disease. Kidney Int. 83(5): 835-44, 2013
- Holden RM, A. Ross Morton, Jocelyn S. Garland, Andrey Pavlov, Andrew G. Day and Sarah L. Booth. Vitamins K and D status in stages 3 to 5 chronic kidney disease. Clin J Am Soc Neph 5(4) 590-7, 2010
- Holden RM et al. Inhibiting the progression of arterial calcification with vitamin K in HemoDialysis patients (iPACK-HD) trial: rationale and study design for a randomized trial of vitamin K in patients with end stage kidney disease. Canadian Journal of Kidney Health and Disease. 2(17): 1-8, 2017
Dr. Hindmarch
- Hemnes AR1, Trammell AW, Archer SL, Rich S, Yu C, Nian H2, Penner N, Funke M, Wheeler L, Robbins IM, Austin ED, Newman JH, West J. Peripheral blood signature of vasodilator-responsive pulmonary arterial hypertension.Circulation. 2015 Jan 27;131(4):401-9; discussion 409. doi: 10.1161/CIRCULATIONAHA.114.013317. Epub 2014 Oct 31.
Dr. James
- Starke, RD, Ferraro F, Paschalaki KE, Dryden NH, McKinnon TA, Sutton RE, Payne EM, Haskard DO, Hughes Ad, Cutler DF, Laffan MA, Randi AM. Endothelial von Willebrand factor regulates angiogenesis. 2011 Jan;117(3): 1071–80.
- Selvam SN, Casey LJ, Bowman ML, Hawke LG, Longmore AJ, Mewburn J, Ormiston ML, Archer SL, Maurice DH, James P. Abnormal Angiogenesis in Blood Outgrowth Endothelial Cells Derived from von Willebrand Disease Patients. Blood Coagul Fibrinolysis. 2017 Oct;28(7): 521-533.