In the final edition of this series focused on providing examples of translational research at the Department of Medicine, I will highlight the activities of Dr. Mark Ormiston and some of my own research. Both of our research programs endeavour to span the gap between the patient and the basic science research laboratory. Although there are many other faculty who run excellent translational research programs, I hope that the examples in this series of three blogs, provides the reader with some sense of translational research in practice. These blogs also highlight the importance of the launch of TIME (Translational Institute of Medicine) as a way of advancing the discipline here at Queen’s University and Kingston Health Science Centre. TIME will also be vital to training the bright young people who will lead our institutions in the future. TIME is a manifestation of the vision that patient care will ultimately be enhanced by offering faculty easy access to state of the art infrastructure operated by superb staff scientists, by mapping research teams and promoting awareness of local expertise and by training graduate students in translation research. TIME is well-aligned with Principal Woolf’s goals of improving the standing of Queen’s University as a nationally leading, research-intensive university. 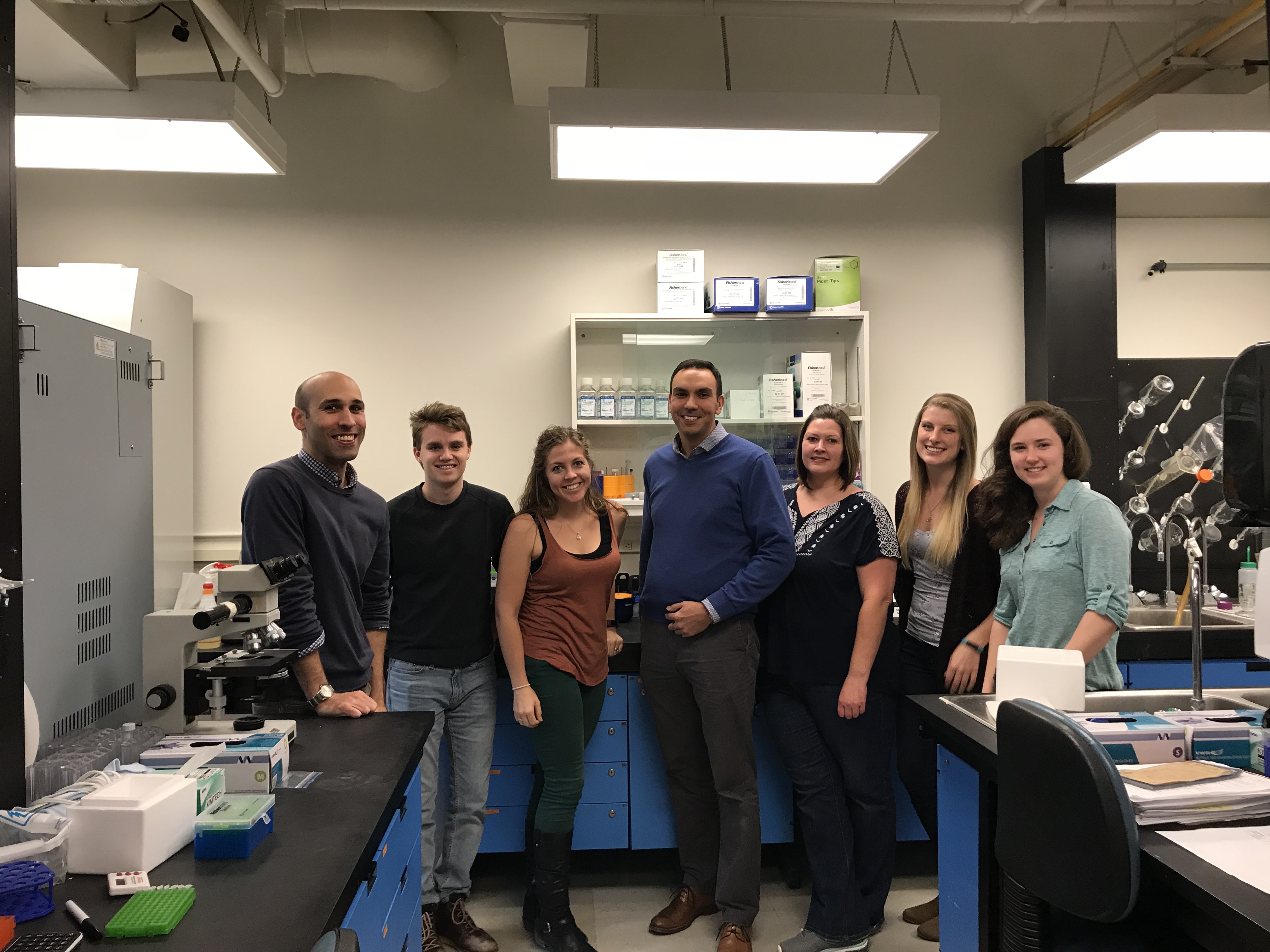
Mark Ormiston (left) and his lab members
The big question: Does immune dysfunction contribute to pulmonary vascular disease? The goal: To understand the contribution of specific immune cells, known as Natural Killer (NK) cells to diseases of vascular remodeling, such as pulmonary arterial hypertension (PAH) Defining the problem: PAH is a fatal disease that preferentially afflicts young women in their 30s and 40s. The disease involves the obstruction of blood vessels in the lung, which leads to a loss of the pulmonary microcirculation, increased stress on the right side of the heart and eventual death due to heart failure. Despite the approval of several treatments, the annual mortality rate for PAH remains high (~15%), in-line with the prognosis of stage III breast cancer. This poor survival is linked to the fact that available therapies primarily treat the symptoms of PAH and do not address the underlying factors driving disease progression. The “Big Idea”: There is a well-appreciated link between PAH and impaired immune function. For example, women with scleroderma (CREST syndrome) and systemic lupus erythematosus (SLE) are particularly prone to PAH. In addition, some viral infections, like HIV, promote PAH. However, there is little understanding of how alterations in an individual’s immune status (called autoimmunity because the body turns against itself) contributes to pathological vascular remodeling. Our research focuses on NK cells, which are best known for their protective effects, including targeting and eliminating cancerous or virally-infected cells. My lab focuses on a novel function of these NK cells, namely their capacity to promote beneficial remodeling of blood vessels. This function is particularly relevant in pregnancy, where NK cells facilitate the expansion of blood vessels in the uterus to enable the delivery of oxygen and nutrients to the developing fetus. We hypothesize that this unique vascular function of NK cells is activated inappropriately in the lungs of PAH patients. Key Research Observations: Our research has identified an impairment of the NK cells from PAH patients. This impairment is linked to a reduction in killing capacity and elevated expression of matrix metalloproteinase 9 (MMP9), an enzyme that is involved in blood vessel remodeling. We suspect that this disease signature is due to chronic exposure of NK cells to an inflammatory protein that is known to be important in PAH, transforming growth factor-b (TGFb). The way forward: We are currently investigating the molecular processes by which the chronic exposure of NK cells to disease factors like TGFb can promote their conversion from a tumor killing cell to one that drives vascular remodeling. We are using cutting edge technology, known as CyTOF mass cytometry, to model this conversion. It is noteworthy that this research would not be possible without a $2m piece of equipment that is available to me through my membership in the Queen’s Cardiopulmonary Unit (QCPU), a new translational research facility at Queen’s University. Mass cytometry uses heavy metal tags to quantify up to 40 protein markers on cells in a mixed population, allowing us to define and distinguish our specific cells of interest. By clustering cells that exhibit a similar marker profile together (see figure), this technology allows us to track the process of NK conversion in the test tube, providing insight into the molecular pathways responsible for this process in the patient. 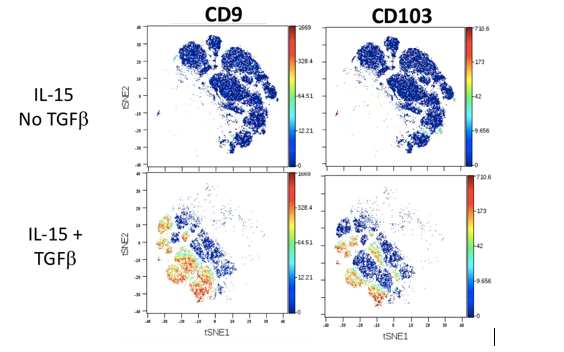
CyTOF analysis of NK cells with and without chronic exposure to TGFb.
NK cells, with and without TGFb, were analyzed by CyTOF mass cytometry and clustered based on the surface expression of 22 protein markers. The intensity of expression for specific markers, such as CD9 and CD103, is indicated by colour, with red indicating a high level of expression. We observe that chronic TGFb exposure is inducing a “uterine-like” induction of CD9 and CD103 in specific subsets within the NK cell population. In the future, we hope to be able to treat PAH by regulating NK cells, either by directly targeting disease-causing NK subtypes or by manipulating the TGFb pathway. Stay tuned!
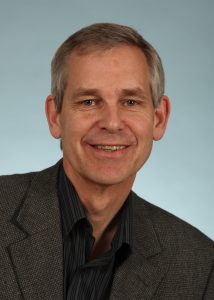
Stephen L Archer MD (Cardiology), Scientific Director QCPU; Professor and Head Department of Medicine (Cardiology):
The big question: Can we target mitochondria to treat pulmonary arterial hypertension (PAH)? The Goal: PAH is a devastating disease in which obstruction of the lung’s blood vessels leads to right heart failure which causes disability and death. PAH occurs in children and adults and is much more common in women than men. We identified two abnormalities of the mitochondria in cells within the wall of the lung arteries in PAH patients. These mitochondrial abnormalities cause lung vascular cells, called pulmonary artery smooth muscle cells (PASMC), to grow very rapidly and block the blood vessel. We believe that this mitochondrial-mediated proliferative phenotype is a central feature of PAH and one which is not addressed by current therapies. By targeting the mitochondrial abnormalities we hope to slow cell growth and restore blood flow to the lung, thereby reducing pulmonary artery pressure and improving right heart function. These goals are similar to Dr. Ormiston’s goals-we are just targeting mitochondria and PASMC instead of NK cells. The “Big Idea”: In PAH, rapid PASMC growth (excess proliferation) and insufficient removal of diseased cells (impaired apoptosis) blocks the lung’s blood vessels. Lung cancer shares this proliferation/apoptosis imbalance. Our idea involves a paradigm shift and views PAH as a disease having similarities to cancer. Thus we plan to attack PAH as a cancer-like disease. Our program exploits two mitochondrial abnormalities, which are shared by PAH and cancer cells, to reduce cell proliferation, enhance apoptosis and thus cure disease. Defining the problem: The 10 drugs that are currently approved to treat PAH are all expensive and none is curative. The average 5-year survival for a patient newly diagnosed with PAH remains poor (50%). Perhaps our failure to cure PAH means we are attacking the wrong target or have an inaccurate understanding of the disease. It is noteworthy that while all approved drugs are largely vasodilators (i.e. they relax constricted blood vessels) only 10% of PAH have vasoconstriction as a major part of the disease. My team is exploiting new discoveries in the areas of mitochondrial metabolism and mitochondrial dynamics to try and understand the relationship between the cell cycle, mitochondrial function and vascular obstruction. We hope to use this knowledge to devise means to eliminate the abnormal cells (or at least slow their growth) and thereby restore blood flow to the lung. Our long-term goal is to develop new treatments for patients with PAH. The Key Research Observation(s): PAH PASMC and cancer cells have abnormal mitochondrial function and structure and we discovered two relevant abnormalities that allow cells to grow too fast and to avoid cell death. They are: 1) impaired oxidative glucose metabolism, with an energetic reliance on glycolysis (so-called Warburg metabolism) 2) increased mitochondrial division (fission). In PAH, mitochondrial metabolism is suppressed because an enzyme called pyruvate dehydrogenase kinase (PDK) is inappropriately activated. PDK inhibits PDH, the main regulator of glucose oxidation. The abnormal cells then process glucose as an energy source solely via glycolysis. Because glycolysis is much less efficient in producing energy than glucose oxidation, PAH cells, like cancer cells, develop a sweet tooth. They consume large amounts of sugar (glucose) to support themselves via glycolysis. Although energetically inefficient, Warburg metabolism takes the mitochondria “off-line” and protects diseased cells from elimination by the body’s main quality control mechanism (mitochondria-mediated apoptosis). Using drugs that inhibit PDK, such as dichloroacetate, we can reactivate PDH, re-engage apoptosis, slow cell growth, and regress PAH (in preclinical models).
The second abnormality relates to mitochondrial structure and the fact that whenever a cell divides its mitochondria must divide in parallel to provide each daughter cell with their fair share of mitochondria. Thus, rapid cell growth (as in PAH and cancer) requires high rates of mitochondrial division, which manifests as mitochondrial fragmentation. Mitochondria normally exist in lengthy networks that extend throughout the cell (think of it like the wiring in a house). However, unlike wiring, the mitochondrial network is dynamic, constantly dividing (a process called fission) and joining together (a process called fusion). In PAH and cancer cells the mitochondria are fragmented (see Figure showing fragmented red mitochondria in human PAH PASMC below). 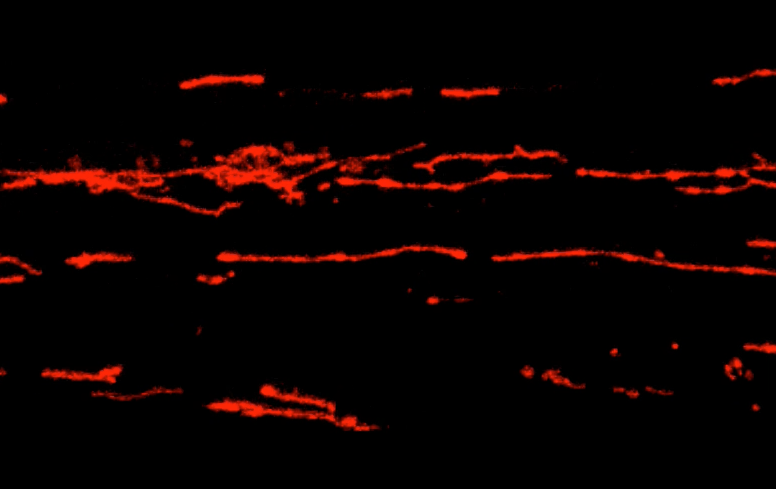
PAH constriction fission and fusion (movie)
Recently we used super resolution microscopy to actually get a picture of the proteins that assemble into a ring and constrict the mitochondria, leading to mitochondrial fission (see below: from Circulation. 2018 Feb 5. pii: CIRCULATIONAHA.117.031258. doi:10.1161/CIRCULATIONAHA.117.031258) Fragmented mitochondria result both from increased rates of mitochondrial fission and impairment of mitochondrial fusion. We showed that the molecular basis for this fragmentation was increased activity of the fission mediator, dynamin related protein 1 (Drp1), and decreased activity of the fusion mediator, mitofusin-2. By inhibiting Drp1 or augmenting mitofusin-2 we can also regress experimental PAH. This is an advance in basic cell biology and preclinical science, but still doesn’t directly translate to improved patient care (i.e. it’s not a complete translational journey).
It’s worth noting that in the course of this voyage of discovery we have created what is both a training ground for the next generation of physicians and scientists and a small business (annual payroll over $500,000 year). I am privileged to lead a team of 5 postdoctoral fellows, a CIP trainee, and 5 staff scientists (see below). We have collaborators at Queen’s University (Dr. Ormiston and Wijeratne in image below) and collaborators in other Universities in Canada, the USA and the UK). Our work has established the benefit of PDK inhibitors, Drp1 inhibitors and mitofusin-2 gene therapy as treatments for PAH and cancer in preclinical models. 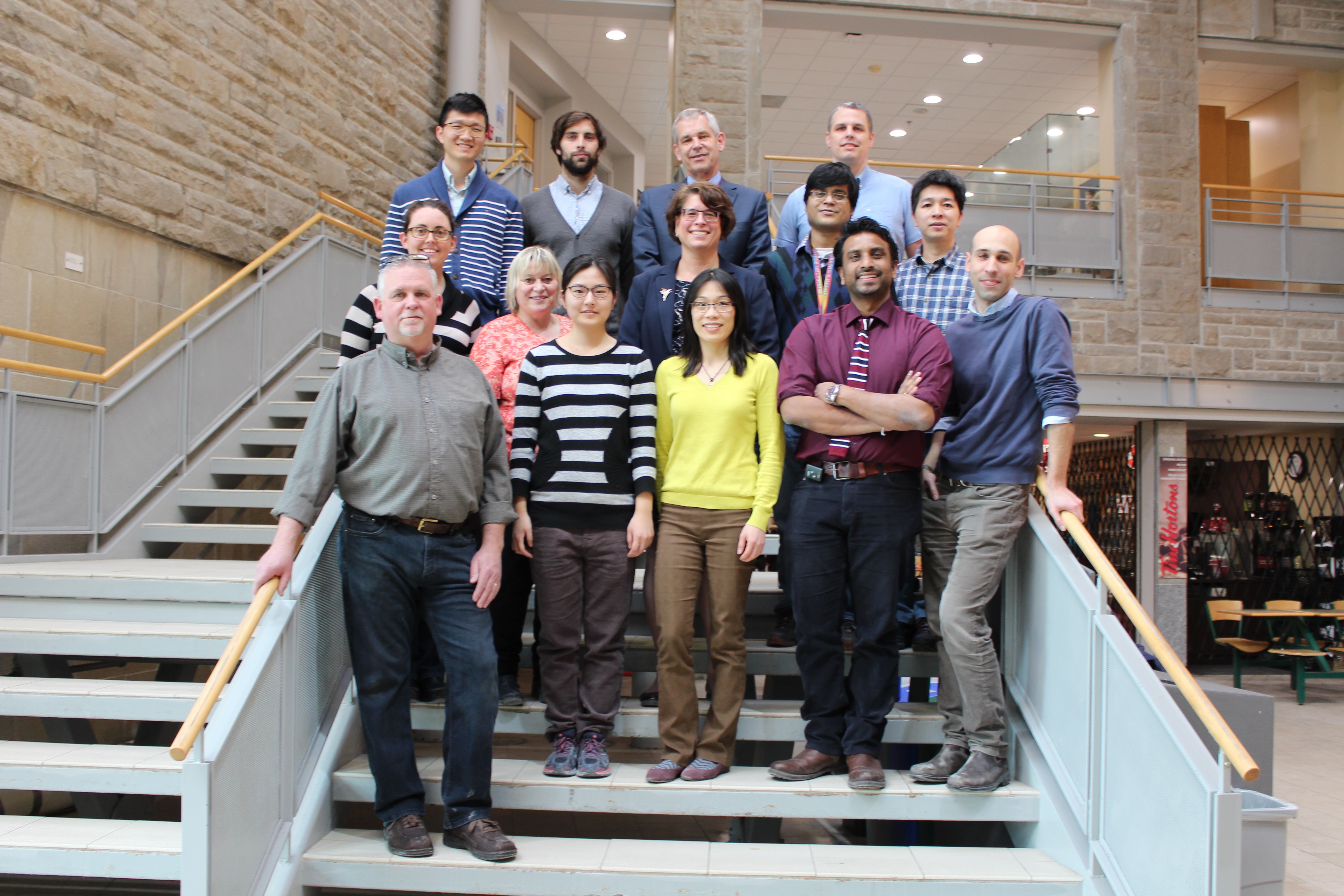
Archer Lab (and friends) 2018
The Way Forward: A colleague (Dr. Evangelos Michelakis, University of Alberta) has taken the next important step in the translational journey. He tested dichloroacetate in patients with PAH and cancer. In two small, Phase 1 studies, referenced below, he showed that the PDK inhibitor, dichloroacetate is safe and may be effective in patients with glioblastoma tumors of the brain or PAH. This illustrates the hand offs that occur in the translational research relay. The most difficult hand-off is from translational scientists, such as Dr. Michelakis or myself, to industry. Only Pharma has the depth of funding required to conduct the large, multicenter research studies that are required to determine whether dichloroacetate or Drp1 inhibitors (or any new therapy) is actually beneficial when added to the current standard of care. The communication between Pharma and Academia is challenging and indeed there is often poor mutual understanding of the goals and priorities of these two solitudes. My lab continues to search for new targets and test new drugs that inhibit Drp1 and PDK as we test our therapeutic paradigm. So, having reviewed limited examples of translational research by faculty from the divisions of Dermatology, Neurology, Hematology, Cardiology and Nephrology, I hope you will agree the time is right for TIME! With the beginning of TIME, we are not only creating a unique network and infrastructure of state of the art equipment, we are also actively recruiting and training the next generation of research leaders. With the launch of the upcoming TIME graduate program, in combination with the TIME infrastructure (in QCPU, GIDRU and elsewhere), we anticipate creating a bustling hub of high-tech, cutting edge research and training that will elevate the quality of research and clinical care at Queen’s University and Kingston Health Sciences Centre. Investment in translational research is a wise investment in our future. Relevant articles:
- Archer, S.L., Mitochondrial Dynamics — Mitochondrial Fission and Fusion in Human Diseases N Engl J Med 2013;
- Archer SL et al Am J Physiol Heart Circ Physiol. 2008 Feb;294(2):H570-8. Mitochondrial metabolism, redox signaling, and fusion: a mitochondria-ROS-HIF-1alpha-Kv1.5 O2-sensing pathway at the intersection of pulmonary hypertension and cancer.
- Michelakis ED et al, Inhibition of pyruvate dehydrogenase kinase improves pulmonary arterial hypertension in genetically susceptible patients. Sci Transl Med. 2017 Oct 25;9(413).
- Epigenetic Dysregulation of the Drp1 Binding Partners MiD49 and MiD51 Increases Mitotic Mitochondrial Fission and Promotes Pulmonary Arterial Hypertension: Mechanistic and Therapeutic Implications.
- Chen KH, Dasgupta A, Lin J, Potus F, Bonnet S, Iremonger J, Fu J, Mewburn J, Wu D, Dunham-Snary K, Theilmann AL, Jing ZC, Hindmarch C, Ormiston ML, Lawrie A, ArcherCirculation. 2018 Feb 5. pii: CIRCULATIONAHA.117.031258. doi: 10.1161/CIRCULATIONAHA.117.031258. [Epub ahead of print] PMID:29431643