We teach medical students, “When you hear hoof beats, think horses”. This is sage advice, designed to focus them on recognizing common diseases. However if the galloping beast has cutaneous stripes…we must acknowledge the possibility it is a zebra.
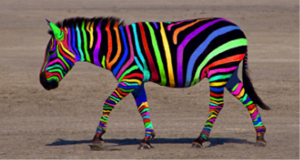
The addition of visual data offers a deeper phenotype. The word phenotype comes from the Greek, phainein meaning "to show", and typos, meaning "type”. Phenotype is defined as: “The set of observable characteristics of an individual resulting from the interaction of its genotype with the environment.”The idea of creating deep phenotypes as a way of recognizing new diseases is top of mind recently based upon exciting discoveries by two young physicians, Dr. Lysa Lomax, at Queen’s University, and Dr. Anna Hemnes, at Vanderbilt University. Dr. Lomax used a deep phenotype approach to discover the genetic basis for a rare epilepsy/myoclonus syndrome, called North Sea Myoclonus. Dr. Hemnes exploited a deeper patient phenotype, that included responsiveness to a vasodilator drug challenge, to develop a blood test that identifies patients with Group 1 pulmonary hypertension (also called PAH) who have excellent long-term prognosis. Physicians, nurses, pharmacists and dentists are busy managing known disease in the “here and now” and may not feel the need to discover yet more diseases. However, unrecognized diseases, with specific causes and cures, are there in the waiting room. They already afflict our patients and the first step toward their discovery and cure is for some bright person (? you) to recognize their unique, deep-phenotype. Once this is done, someone (? you) can collect a homogenous cohort of patients that share the same disease features and then (often through collaboration with basic scientists) apply genomic and proteomic tools to identify the molecular cause of the disease. This new disease will usually have its own genetic, epigenetic or acquired cause and this recognition may suggest a customized treatment, so called personalized medicine. In some cases the disease identified will actually be entirely new, but in many cases deep phenotyping takes a broad syndrome (like epilepsy or pulmonary hypertension) and parses it into several more uniform diseases, linked by a unified cause. Remember that all edema was once dropsy…now we have systolic and diastolic heart failure, each with deep phenotypes that relate to molecular mechanisms (think hypertrophic cardiomyopathy due to myosin heavy chain mutation).
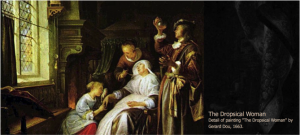
Disease phylogeny is of more than academic interest. Think of Gregor Mendel, who inferred human genetic inheritance by observing the deep phenotype (colour) of the common pea plant to identify the process of genetic inheritance! In case you missed genetics 101 (or, like me, just failed to understand it)…check out this video:
What do I mean by deep phenotype? By deep I mean detailed. This means taking the classical characteristics of the disease (the signs and symptoms obtained from a history and physical examination), and augmenting them with the imaging and molecular traits to obtain a more fulsome explanation of the disease. Thus a deep phenotype offers the possibility of a more clear identification of the molecular basis for specific disease features and thus the possibility of more specific or personalized cures. To see deep phenotyping in action let’s review the discovery by Dr. Lysa Boissé Lomax and colleagues. She recently gave a superb Medical Grand Rounds at Queen’s University on the discovery of a very rare new disease with the intriguing name: North Sea Myoclonus.
Lysa’s voyage of discovery began with a fellowship trip to Australia where she worked at the Brain Institute in the University of Melbourne (http://www.melbournebraincentre.edu.au) with epilepsy genetics pioneers, Drs. Sam Berkovic and Ingrid Scheffer. Lysa and her mentors were interested in patients with a particular phenotype of epilepsy that is admittedly rare (<1% of all epilepsy). Their patients comprised a subset of a rare epilepsy syndrome: Unverricht-Lundborg Disease. These patients have 1) Myoclonus (sudden, involuntary jerking of a muscle or group of muscles) 2) Generalized tonic clonic seizures (generalized seizures characterized by sequential, involuntary, muscle contraction and relaxation) 3) Progressive neurological decline (Progressive dementia and ataxia) Half of the cases of Unverricht-Lundborg Disease result from homozygous mutation of the cystatin b gene (the findings from earlier deep phenotyping studies). This gene, when mutated, seems to allow the death certain GABA-ergic neurons and thus causes myoclonus and seizures. However, Lysa and colleagues recognized that almost half the cases of Unverricht-Lundborg Disease had no mutation of the cystatin b gene. Lysa and the Brain Institute team next identified 159 such patients and proceeded to perform deep phenotyping (by analyzing clinical history, family history, physical examination, neurophysiology, imaging, pathology). Phenotype 1: The North Sea Myoclonus phenotype:
Her patients lacked the cystatin b mutation and had a severe phenotype: rapid progression of the myotonia and seizures, leak of a muscle enzyme called CK, difficulty speaking (dysarthria) and swallowing (dysphagia) and ultimately died at a young age (24 years on average). Many had musculoskeletal malformations, like the severe scoliosis seen in the X-ray of an afflicted person (above). These patients paradoxically worsened when they received common epilepsy treatments, such as phenytoin or carbamazepine. Their deep phenotype included highly photosensitive patients who had juvenile tonic clonic seizures as well as myoclonus, minimal cognitive decline and a normal electromyogram. Phenotype in hand its just a matter of sotring through the 25,000 human genes to identify a cause! They chose to use linkage analysis, a technique that identifies the chromosomal location of an unidentified disease-causing gene by tracking its known “neighbours” (satellite markers). Linkage analysis combines a deep phenotype with careful family history and monitoring the inheritance of these satellite markers as they pass through the family tree, see genome family tree.
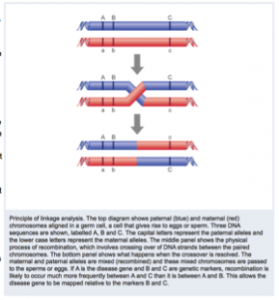
As nicely described on this genome.wellcome website, “disease genes are mapped by measuring recombination against a panel of different markers spread over the entire genome. In most cases, recombination will occur frequently, indicating that the disease gene and marker are far apart. Some markers however, due to their proximity, will tend not to recombine with the disease gene and these are said to be linked to it. Ideally, close markers are identified that flank the disease gene and define a candidate region of the genome between 1 and 5 million bp in length. The gene responsible for the disease lies somewhere in this region.” Alternatively the research team could have jumped straight to whole exome sequencing, a newer technique that directly confirms the sequence of all genes. However, linkage analysis is a nice way of identifying candidate genes that are linked to the disease and so they chose to do this and then ultimately confirmed the identity of the imputed gene using exomic sequencing.
Linkage analysis suggested the causal gene might be one called Membrin (also known as GOSR2). The Membrin gene encodes a snare protein in the Golgi apparat
us.They then sequenced this unexpected candidate gene and found a mutation in the myoclonus patients (a guanine (G) was replaced by a thymidine (T) at position 430 (430G>T)). This gene mutation results in a change in the protein sequence from a glycine at amino acid 144 in the protein to a Tryptophan, which somehow creates a dysfunctional Membrin protein and somehow leads to this horrible syndrome. Based on the fact that many of the patients originate from locations around the North Sea they called the disease North Sea Myoclonus-although perhaps it is better called membrin-mutation myoclonus! (Brain. 2013 Apr;136(Pt 4):1146-54. doi: 10.1093/brain/awt021. Epub 2013 Feb 28.) (http://tinyurl.com/oqnyvsl)
This discovery (which resulted from arduous work) resulted in a simple test that can now be ordered from a doctor’s office. There are now diagnostic panels (such as the one below) in which GOSR2 or Membrin mutations (circled in red) can be identified, along with 69 other known genetic causes of epilepsy! The main challenges of such testing are financial (cost to patients or payers) and ethical (there is always a need for genetic counselling).
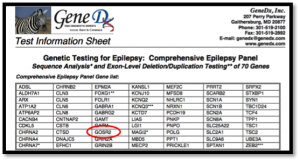
Phenotype 2: Pulmonary hypertension patients who respond to vasodilator drugs and have long survival 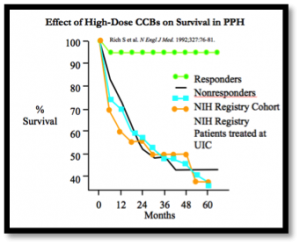
Pulmonary arterial hypertension (PAH) is a lung vascular disease in which the blood pressure in the lung circulation (called the mean pulmonary artery pressure) is elevated to over 25 mmHg. The rise in pressure is due several factors: blood vessel contraction (called vasoconstriction) and blood vessels blockage or destruction, due to excessive cell growth (proliferation) and insufficient cell removal (apoptosis), as reviewed in Circulation. 2010; 121: 2045-2066. The World Heath Organization (WHO) has a classification system for pulmonary hypertension (PH), recognizing 5 types. However, it is clear that within even WHO Group 1 pulmonary hypertension there are likely dozens of discrete diseases, each with their own deep phenotype. Despite 9 approved drug treatments, half of all PAH patients die within 5-years of diagnosis. However, Dr. Stuart Rich identified a subset of PAH patients that has a much better prognosis (see graph above). This group (approximately 10% of PAH patients) responds to the administration of a blood vessel relaxing drug (such as inhaled nitric oxide or a calcium channel blocker, such as nifedipine) with a marked drop in mean pulmonary artery pressure and a rise in cardiac output. These patients usually live many years.
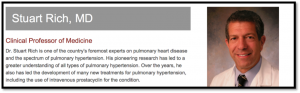
We have long suspected being a Vasodilator Responder (VR) is a deep phenotype that may identify a new subtype of Group 1 pulmonary hypertension. A team at Vanderbilt University, Queen’s University and the University of Chicago wanted to identify the molecular basis of this phenotype using a blood test, in hopes of identifying patients with a better prognosis and that could be treated with a cheap and effective therapy, such as nifedipine. The team was led by Dr. Anna Hemnes (below), a lung specialist and clinician-scientist at Vanderbilt University in Nashville Tennessee.
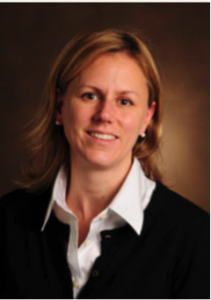
Although vasodilator responsive PAH (VR-PAH) accounts for a minority of cases, they have a pronounced response to inexpensive drugs called calcium channel blockers as well as better survival (as compared to non-responsive PAH patients (VN-PAH). Anna and team (I am a proud team member) performed microarrays on blood cells (cultured lymphocytes) from VR-PAH versus VN-PAH patients. She used quantitative PCR to measure gene expression rather than gene sequence (a difference from Dr Lomax’s approach) By identifying the 25 most differentially expressed genes between the VR-PAH and VN-PAH cases she was able to build simple decision trees to identify the vasodilator responsive group. Ultimately it proved that one need only measure the expression levels of two genes: DSG2, a desmosomal cadherin involved in Wnt/β-catenin signaling, and RHOQ, which encodes a cytoskeletal protein involved in insulin-mediated signaling. These decision trees (shown in the figure below) correctly identified the vasodilator-responsive vs. vasodilator-nonresponsive patients both at Vanderbilt and the University of Chicago.
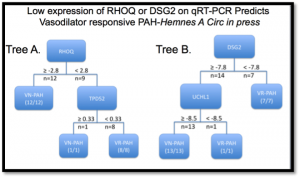
These differences, just published on-line in the journal Circulation, October 2014, may reflect the existence of different stages of a single disease (PAH) or perhaps two discrete pulmonary hypertensive diseases. In either case the different phenotypes merit different treatments and have different prognosis, permitting physicians to approach these patients and offer a personalized medicine strategy. Her PAH discovery is in keeping with a recent position paper from the American Thoracic Society (ATS) on how to use deep phenotypes to guide PH research. The senior author, Dr. Raed Dweik at Cleveland Clinic noted, “Accurate phenotyping will advance understanding of mechanisms, which can be used to guide targeted management strategies.“Am J Respir Crit Care Med Vol 189, Iss 3, pp 345–355, Feb 1, 2014 My colleague at Vanderbilt Dr. John Newman, Elsa S. Hanigan Chair in Pulmonary Medicine, (seen below) is a PH expert. He reminded me that the US NIH has created an entity to promote just the type of phenotyping and disease discovery that I have described in this blog.
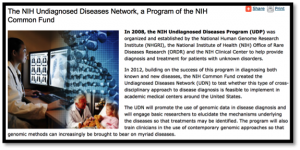
Physicians are increasingly positioned to create a deep phenotype of diseases, find their cause and help create personalized treatments. This deep phenotyping allows us to paint a picture based on the history and physical examination and embellished by the findings from biomarkers assays and molecular imaging studies. Many treatment failures and adverse drug responses today likely result from applying a treatment to “the wrong disease”. We need to teach trainees to be masters of phenotyping who can sort patients by their deep phenotype into increasingly personalized and smaller, more tightly defined disease groups.